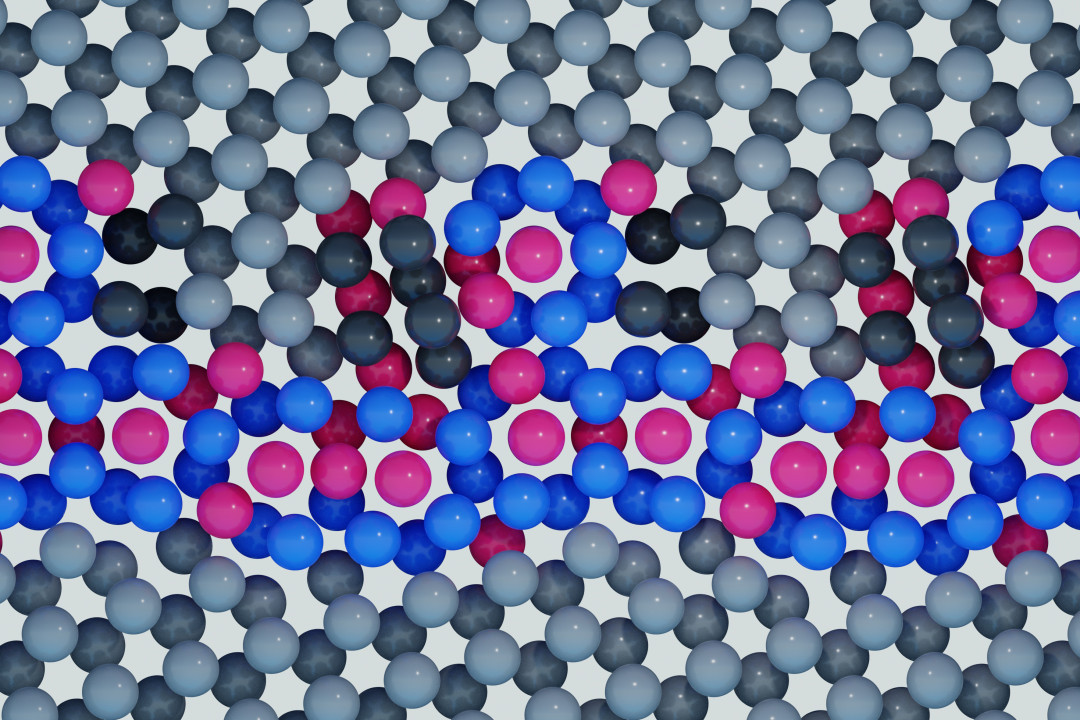
Using state-of-the-art microscopy and simulation techniques, an international team of researchers has systematically observed how iron atoms change the structure of grain boundaries in titanium. They were surprised to discover that ‘iron atoms not only accumulate at the interface, but also form completely unexpected cage-like structures,’ explains Prof. Dr. Christian Liebscher from the Research Center Future Energy Materials and Systems of the University Alliance Ruhr. The researchers had not expected such behaviour. Their results were published in the journal Science on 25 October 2024.
Most engineering materials are polycrystalline: they are made up of different crystals, in which the atoms are arranged in a regular lattice. These crystals are not aligned in the same way everywhere, and the interfaces that separate them are known as grain boundaries. ‘These grain boundaries have a huge impact on a material's durability and overall properties,’ says Dr Vivek Devulapalli, who carried out the microscopic work for the study. He adds: ‘But we have a very limited understanding of what happens when elements attach to grain boundaries and how they affect a material's properties.’
The key to success was the observation and modelling of the structures at atomic resolution. The researchers correlated their results from atomic-resolution scanning transmission electron microscopy with advanced computer simulations. A new algorithm for predicting the grain boundary structure was able to generate the experimentally observed structures, thus enabling the investigation of their structure. ‘Our simulations show that for different iron contents, we always find the cage structures as the underlying building blocks of the different grain boundary phases. The higher the iron content at the grain boundary, the more icosahedral units appear and eventually agglomerate,’ explains Dr Enze Chen of Stanford University. An icosahedron is a geometric object with twelve corners or vertices, which in this case are occupied by atoms, and 20 planes.
‘We have identified more than five different structures or grain boundary phases of the same interface, all of which consist of different arrangements of the same icosahedral cage units,’ adds Dr Timofey Frolov, who led the calculations for the study.
A closer look at the cage structures revealed that the atoms adopt an icosahedral arrangement, with the iron atoms at the centre of the icosahedron and the titanium atoms at its corners. ‘The icosahedral cages enable a dense packing of iron atoms, and since they can form aperiodic clusters, more than two to three times the amount of iron can be accommodated at the grain boundary,’ explains Vivek Devulapalli. ‘It looks as if iron is trapped in quasicrystalline grain boundary phases,’ adds Chen. “This is attributed to the properties of the icosahedral cages,” says Liebscher, ’and we now have to find ways to investigate how they influence the interface properties and thus the material behaviour.’
Understanding and controlling the formation of icosahedral grain boundary phases with different structures and properties can potentially be used to tailor the properties of materials themselves. The researchers now want to systematically investigate how these novel grain boundary states can be used to influence material behaviour, tune a particular material functionality and make materials more resistant to degradation processes.